[ad_1]
Buildings of the future will be grown on-site, says Wil Srubar. They’ll be made from hemp, or algae or specially engineered wood — or bacteria that can photosynthesize, like the cyanobacteria mortar he and his research team recently grew between a lattice of sand and water-based gel.
“In my future world, we don’t burn limestone to make cement, and we don’t melt sand to make glass,” he says. Instead, essential building materials might be grown from bacteria that glow (bioluminescence) or from engineered lichen, so that walls are infused with the organism’s remarkable properties. Imagine a house that could filter carbon dioxide from the air or change color if harmful gases are present.
This may sound fanciful, but the work is already underway. Srubar, an assistant professor of architectural engineering at the University of Colorado Boulder, runs the Living Materials Laboratory, where he and his colleagues fiddle with living bacteria to create building materials that grow themselves.
Srubar grew up outside of Houston, on a cotton farm and cattle ranch, “where things were very much alive.” Buildings, in contrast, were “so static and nonliving,” he says. The skyline of Houston inspired him to become a structural engineer, but he wanted to do it differently. “Why can’t we bring building materials to life?” he says.
The construction industry — from the mining and smelting of raw materials to dealing with the waste from demolished structures — has a huge environmental footprint that is often overlooked. It produces 11% of global greenhouse gas emissions. That’s a staggeringly high number, four times the emissions of the whole aviation sector. Added to this, the United Nations predicts 2.3 billion more people will live in cities in 2050 than do now. Construction will have to expand rapidly to keep pace.
But in that same period, greenhouse gas emissions must be cut sharply; emissions must drop 7.6% every year this decade to hold warming below 1.5 degrees Celsius (2.7 degrees Fahrenheit) — or 2.7% each year to hold it below 2 degrees C (3.6 degrees Fahrenheit) — according to the U.N. The global scientific community considers warming beyond 2 degrees C to be catastrophic, but crisis conditions could arise from levels of warming lower than that.
For Srubar and a growing group of material scientists and designers like him, the increasing need for more buildings is a threat to sustainability but also an opportunity to do things very differently. The consequences of failing to change the industry are just too high to ignore ― revolutionary ideas are badly needed to stave off catastrophic climate change.
A Concrete Problem
The vast majority of construction’s huge carbon footprint comes from concrete ― the most widely used substance on Earth after water. Chances are, if you’re reading this in a multi-story building, you’re encased in concrete right now — or at least sitting atop a concrete foundation. Sidewalks are concrete, bridges are concrete, warehouses are concrete.
The ubiquitous material is strong and resilient, but producing it is extraordinarily harmful to the planet. It accounts for about 8% of global greenhouse gas emissions.
Humans have been making concrete since ancient Roman times. Roman concrete, which was made with volcanic ash, enabling it to grow harder as it took on moisture, was so tough that many of their cement structures are still around today.
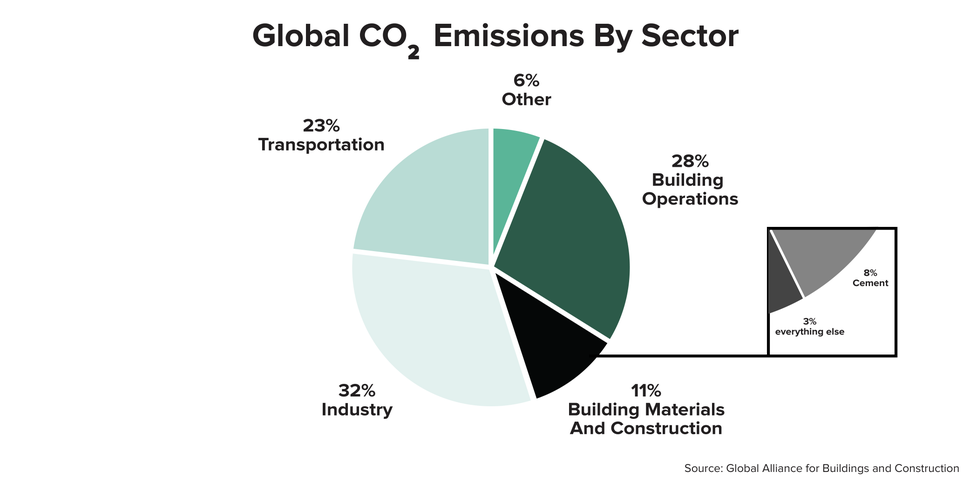
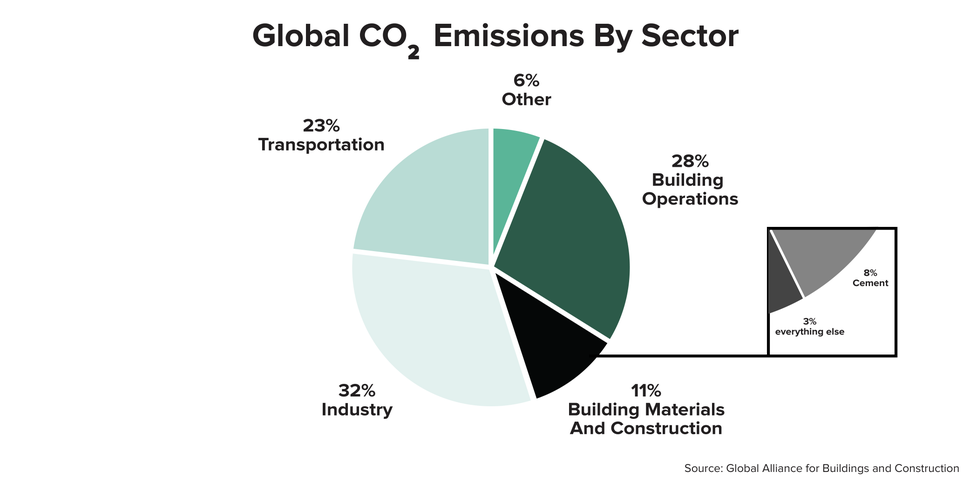
Modern cement — the active ingredient in concrete — is made primarily from limestone, which is heated with other raw materials in a kiln at more than 2,600 degrees Fahrenheit. Limestone is mostly calcium carbonate. The intense heat breaks apart the molecule, burning off carbon dioxide as a gas and leaving calcium oxide behind, which coalesces into gray marble-sized spheres called clinker.
The clinker is cooled and ground into a powder that hardens phenomenally well when combined with different amounts of water and an aggregate, like sand. The final product is known as concrete.
Making clinker produces the vast majority of the emissions associated with the final concrete product. In addition to the CO₂ released from the kiln, just getting the kiln that hot takes a lot of fuel. And cement companies often burn the dirtiest ad-hoc fuels to fire their kilns, like old tires. “The U.S. is big on that,” says Sabbie Miller, a materials scientist at the University of California, Davis. “We use a good amount of tire burning, as well as pet coke,” she says of the extremely high-carbon waste product from the oil refining industry.
If cement manufacturing were a country, it would have the third-highest carbon emissions in the world. It emits more pollution than all the trucks in the world. While the climate effects are astronomical, the health effects are also dangerous. In addition to carbon dioxide, cement production emits sulfur oxides and nitrogen oxides, which are precursors to ozone ― better known as smog. It also spews fine particulate matter into the air. Both ozone and particulate matter are linked to a long list of health problems, including asthma, lung cancer and developmental delays in children.
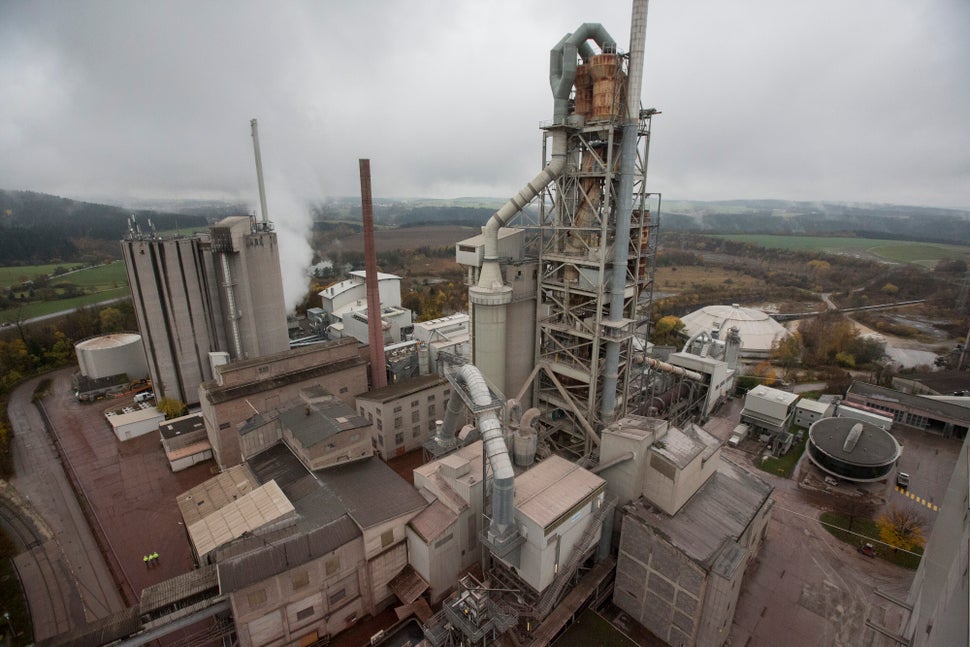
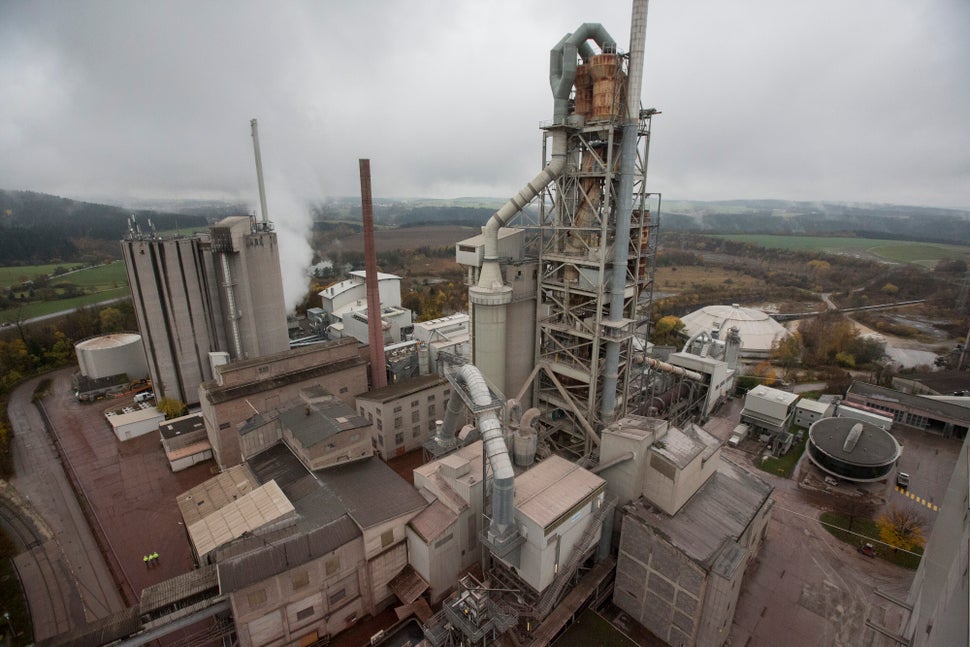
“Currently, [these] external costs of concrete production from climate change and air pollution are comparable to the total market value of the industry,” wrote Miller along with her colleagues in a paper released last month in the journal Nature Climate Change.
Then there is the issue of sand ― by weight, it is the biggest component of concrete. Each ton of cement is combined with 6 or 7 tons of sand or gravel; the cement is the structural “glue” that holds the sand together. Sand mining can have devastating repercussions for communities, both due to land erosion as well as from air pollution. Illegal sand mining is common, leading to unchecked environmental destruction. At least 24 small Indonesian islands had disappeared by 2010 due to sand mining.
Concrete may have an outsized environmental impact, but it’s far from the only problematic construction material.
Just as concrete dominates the outer structure of our built environment, gypsum drywall has become the default for our inner walls. Gypsum is a naturally occurring mineral composed of calcium, sulfur and oxygen, and it is incredibly abundant ― the U.S. alone has more than 55 gypsum mines. When pressed between sheaves of paper, it makes an excellent, customizable, naturally fire-resistant and incredibly cheap wall material. Since skyrocketing in popularity after World War II, gypsum drywall has become ubiquitous in U.S. homes.
But the incredible utility of drywall is not without its costs. Workers in gypsum mines and on construction sites may inhale gypsum and silica dust, which can provoke a range of respiratory diseases and can increase the risk of silicosis and lung cancer.
Respiratory conditions can also make a person more susceptible to the worst effects of lung infections, like COVID-19, while air pollution can make a person more likely to contract viruses in general.
Unlike steel and wood, which are recycled from construction products at a high rate, almost no gypsum drywall gets recycled. When drywall gets wet or dirty, it is almost impossible to use again, and it crumbles easily, making it hard to reuse. Roughly 13 million tons of drywall is generated as waste in the U.S. per year, of which about 2% is recycled.
That means nearly all gypsum drywall winds up in landfills, where it can get wet. Wet gypsum produces hydrogen sulfide, a gas that smells like rotten eggs. Not only is it a nuisance, but the gas also poses potential health risks to communities near landfills. Hydrogen sulfide gas is toxic at high concentrations. Some communities in Canada won’t even accept drywall at landfills due to concern over hydrogen sulfide. Hydrogen sulfide runoff, meanwhile, can contaminate water, threatening wildlife.
Concrete and drywall are just two parts of the vast, complex ecosystem of construction materials. Both are miracle materials but also outsized threats to sustainability.
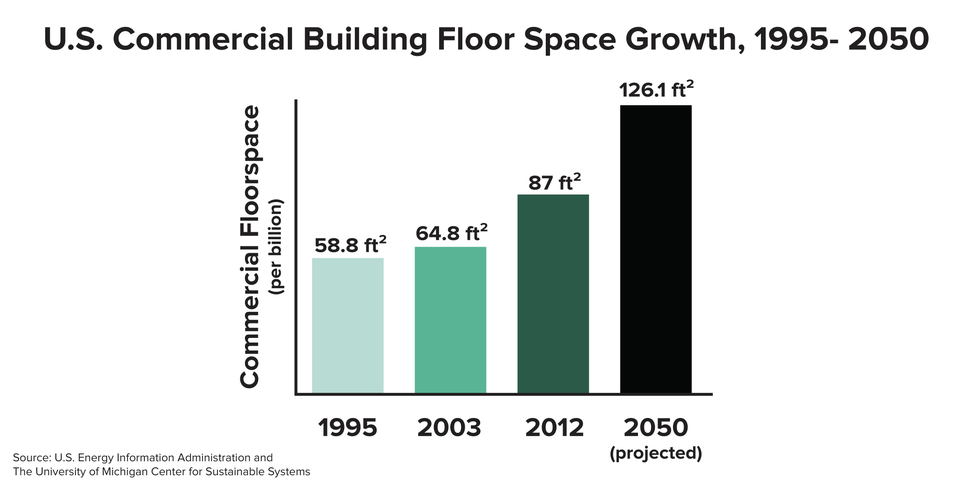
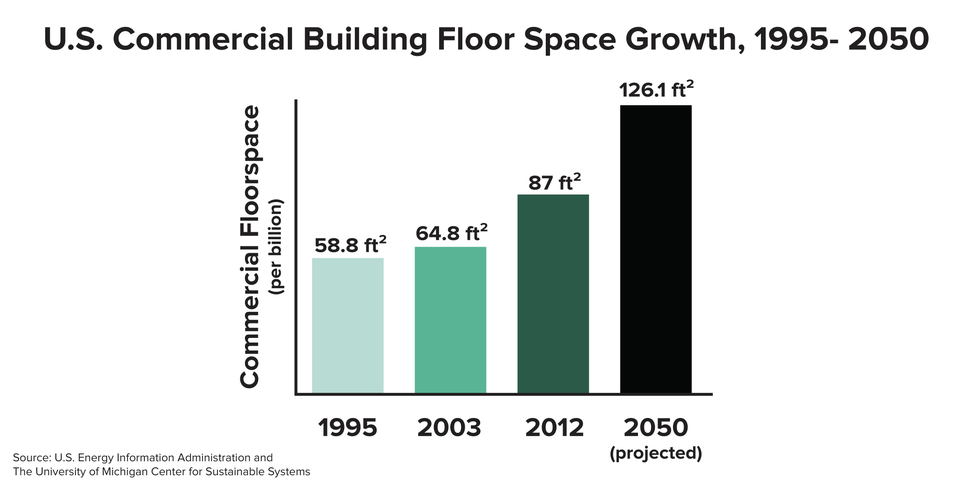
Construction is predicted to grow as urbanization increases across the world, especially in Southeast Asia and sub-Saharan Africa. By 2060, the U.N. projects the international construction community will add 2.5 trillion square feet of buildings, which is equal to the world’s entire current building stock. That rate of addition is the equivalent of adding an entire New York City to the planet every 34 days for the next 40 years.
Cement production is projected to increase to over 5 billion tons over the next 30 years. Yet, to achieve the goals set out by the Paris climate agreement, annual emissions from the cement industry would have to fall by at least 16% by 2030.
Sustainable Solutions
There are solutions. Miller and her colleagues identified a range of promising changes to make to the concrete industry that collectively could reduce its climate and health damages by 44%.
Not all of those solutions are immediately available, however. For example, the team included carbon capture technologies, which would use tools to capture carbon emissions before they have a chance to be released into the atmosphere, that are unlikely to be deployable for five to 25 more years.
But others are available now. One simple improvement would be to stop using ultra-dirty fuels to heat kilns; just replacing old tires and other low-quality fuel with conventional oil would reduce greenhouse gas emissions of concrete by 9% and could reduce the health damages associated with air pollution by 14%. So could reducing the proportion of clinker to each batch of concrete. Using other materials as aggregate — like salvaging glass from waste streams and grinding it into a fine powder — would replace the amount of cement needed per each batch.
Substituting some clinker for other materials can be deployed cheaply and immediately, according to a report from the British policy institute Chatham House, because it doesn’t require new equipment. “It is, therefore, especially important to scale up clinker substitution in the near term while more radical options, such as the introduction of novel and carbon-negative cements, are still under development,” the report’s authors state.
One of the biggest obstacles, though, says Miller, is the industry’s hesitation to try new things. “It’s a really slow-moving industry.”
Where Miller sees hope for more immediate solutions is in the design phase ― not production. Designers and structural engineers, she says, could have tremendous power to change the equation by designing buildings to use less concrete in the first place. “Engineers do have a huge amount of control over how designs are being specified. Just the smallest changes can have a huge benefit.”
Kate Simonen, an architect and structural engineer at the University of Washington, agrees.
We need to think about not just what materials we use but how we use them and what we choose to build, says Simonen, who is also the director of the Carbon Leadership Forum, a 10-year-old consortium of building experts pushing for the decarbonization of the construction sector.
“Don’t tear down buildings and rebuild them if you can reuse them,” she says. Although it’s often a lot less labor-intensive to demolish and rebuild, rather than to retrofit an existing building for a new use, says Simonen, there is a tremendous climate cost to using all-new building materials.
She thinks there’s a role for near-term solutions, such as making the materials that already exist more efficiently, as well as pushing ahead on the longer-range development of materials like the cyanobacteria bricks.
Designers and researchers across a range of disciplines are currently chasing a slew of solutions to bring down the “embodied carbon” in buildings ― meaning the carbon emissions attributed to the manufacturing of building materials.
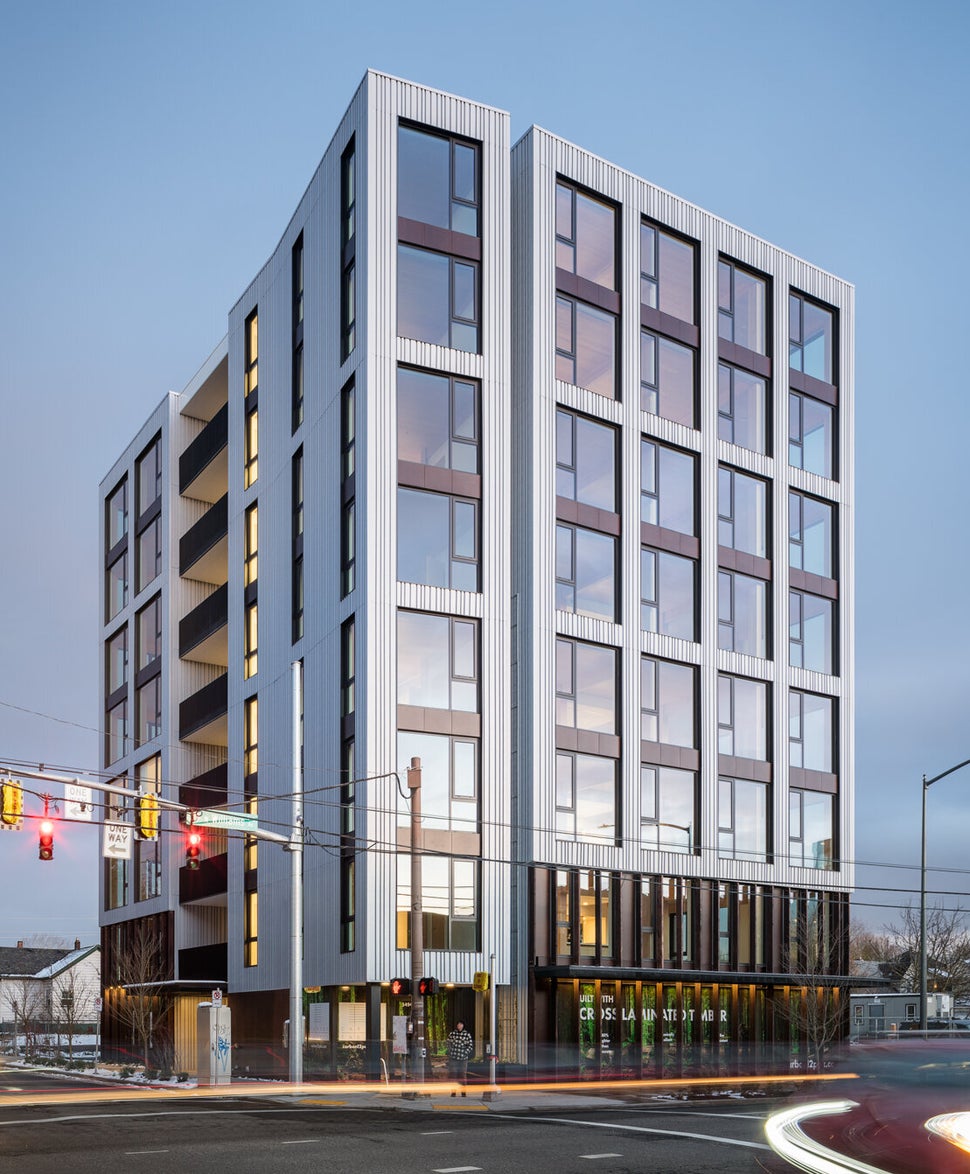
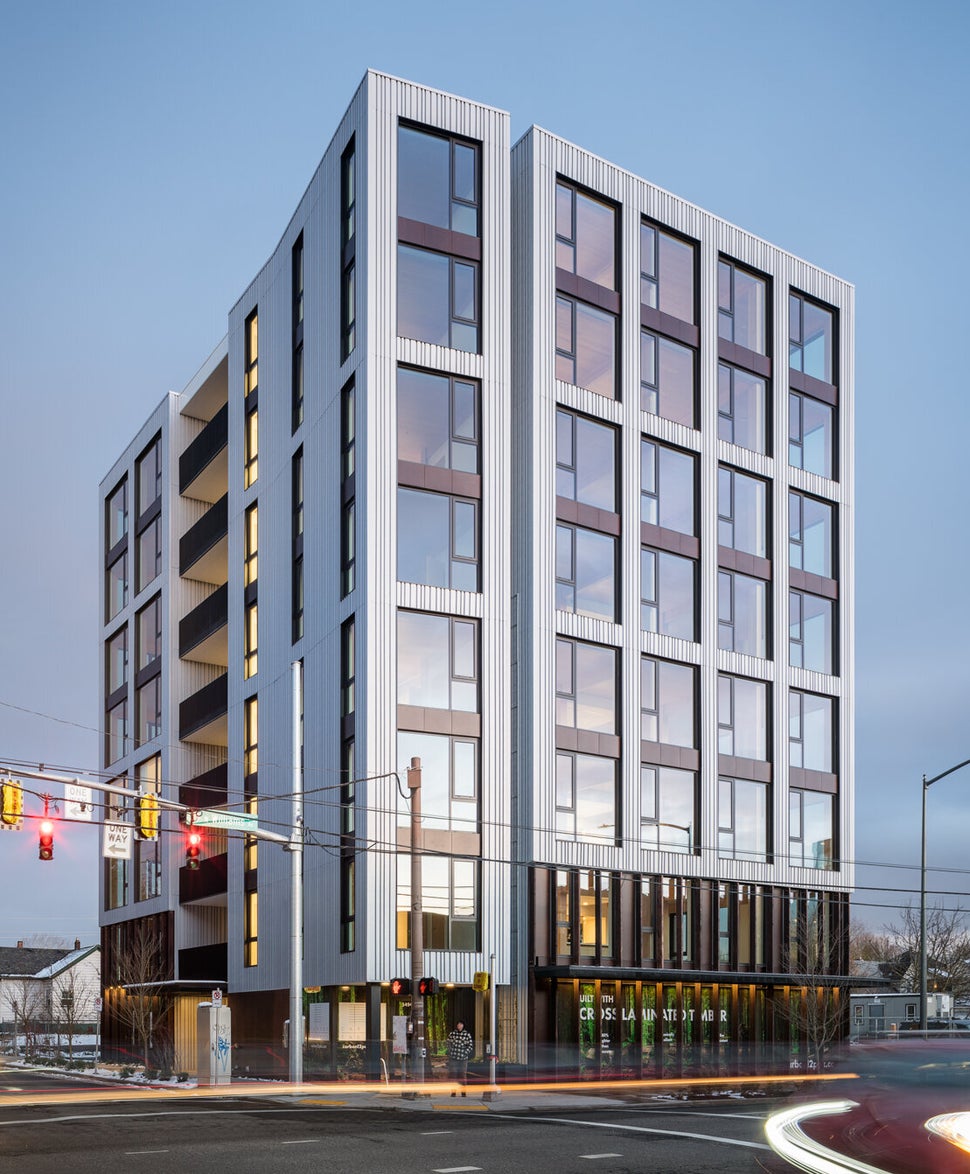
Among those techniques, “mass timber,” an approach using ultra-strong engineered wood as a replacement for reinforced concrete in buildings, probably comes up the most among architects and designers ― the potential for wooden skyscrapers is an extremely hot topic. An 18-story mass timber building went up in Vancouver, Canada, in 2016, and in Norway, an 18-story tower opened last month.
There is growing support for this in the U.S., where several projects are already in the works. At the moment, however, wood buildings in the U.S. can’t legally rise past a six stories ― Carbon12, an eight-story building in Portland, Oregon, is currently the tallest all-wood building in the country. But in 2021, building codes are set to be updated to permit high-rise wood buildings up to 18 stories.
Proponents of mass timber say that using sustainable forestry methods to grow enough wood to replace concrete in buildings could have a greater climate benefit from solely planting trees because it would lock in place the carbon inside the trees, rather than it being released, as happens when a tree dies and decomposes, or burns in a wildfire. Plus, the emission savings from using wood instead of concrete would be formidable. In other words, cities of the future could be transformed from carbon sources to carbon sinks, simply by being built with engineered timber.
But drawbacks remain: There is unlikely to be enough wood in the world to construct wood buildings on a mass scale. And it remains to be seen if forestry can truly be made sustainable enough for this to be a helpful, rather than harmful, approach. The carbon-sink argument applies only to new forests; old-growth forests that have been standing for hundreds of years are excellent long-term carbon sinks and are extremely vulnerable to being lost.
Other innovations involve using agricultural products as building materials. In certain circles, support is growing, albeit slowly, for “hempcrete,” a building material made from hemp. Hemp has been used as a traditional building material in Europe for thousands of years. The plant grows quickly, unlike wood, and uses far less fertilizer than traditional crops, such as corn. Hemp’s fibers mix readily with lime to create a strong, light concrete. It doesn’t mold, and it’s naturally pest-resistant. There are still relatively few hemp buildings in the U.S.; the first was built in California in 2010, and as of 2018 there were 50 homes made with hempcrete, according to a New York Times report. But that could soon change: The most recent farm bill in the U.S., signed into law in 2018, permitted industrial hemp farming in the country for the first time.
One benefit of using plants is the potential to grow your building material nearby, eliminating international supply chains. “I see a great potential of reimagining local supply chains,” Simonen says. For example, she asks, “where is there a lot of agricultural waste, and how can we turn it into a building material?”
In Europe, commercial-grade wall panels are being made out of pressed straw and are being installed in multi-story buildings. A company in California is making rice husks into pressed-board panels; rice farming produces a lot of unusable straw that often gets burned as waste or thrown into waterways and landfills to rot, both of which produce carbon emissions. By pressing the husks into a building material, that carbon stays locked up.
For now, these solutions are small. And while these niche solutions could eventually scale up, those dreaming of a concrete-free world should keep their feet on the ground.
“Concrete is incredibly useful. You can put it into contact with the ground, it doesn’t rust or wash away. It can also use a lot of local materials,” Simonen says, making it an equitable material in the sense that no single country has a monopoly on its production. “The goal of eliminating use of cement is not a realistic position.”
“We need to be more intentional about everything we build, instead of focusing on eliminating one material. All materials have their place,” she says. Instead, the industry must focus on making building materials more efficient and using them more thoughtfully, and only in quantities that are absolutely necessary. “We shouldn’t use them willy-nilly,” Simonen says.
As for “living” materials, like Srubar’s cyanobacteria bricks, these are visionary yet far-off solutions.
In one study, published in October, Srubar and his colleagues genetically programmed E. coli to create limestone particles of different shapes and toughness that could, in theory, be used to develop a novel building material.
And in his team’s latest work, published in March, they demonstrated that not only could they grow bricks from cyanobacteria, but they could keep them alive and prompt them to reproduce. One brick would grow into two, which would grow into four. “We were toying with the concept of exponential manufacturing,” he says. At certain temperatures, the bacteria were dormant, “like how you put yogurt in the fridge to prevent bacteria growth,” he explains. But change the temperature or humidity, and “you can certainly bring them back to life to self-heal or grow another generation of bricks.”
Right now, he says, the final product is literally green, thanks to the color of the cyanobacteria itself, and has the load-bearing capacity of typical mortar (think of the concrete between bricks on a building). With more work, he hopes to increase its strength to that of a structural brick. “We’re just scratching the surface with what the living bacteria would do.”
And while solutions like these are years away and have yet to make it out of the lab and into real-world application, they paint an alluring picture of a future where building cities is no longer an existential threat to the habitability of the planet.
For more content and to be part of the “This New World” community, follow our Facebook page.
HuffPost’s “This New World” series is funded by Partners for a New Economy and the Kendeda Fund. All content is editorially independent, with no influence or input from the foundations. If you have an idea or tip for the editorial series, send an email to thisnewworld@huffpost.com.
Calling all HuffPost superfans!
Sign up for membership to become a founding member and help shape HuffPost’s next chapter